Jiguata Project
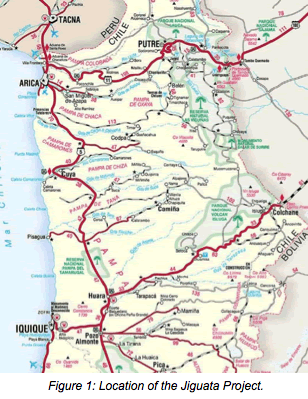
Jiguata is located approximately 150 km east-northeast of the city of Iquique in Region I of Chile. Access is straightforward, being a 3-hour drive from Iquique. The small settlement of Lirima lies 7 km to the southwest and the project lies at an elevation of between 4,600 and 4,700 m (~15,000 feet) above sea level (Figure 1).
Exploration Concessions
Minera Catalina SA applied for its first Jiguata exploration concessions (“pedimentos mineros”) in July 2010. In Chile, exploration concessions have a two-year life from their date of approval.
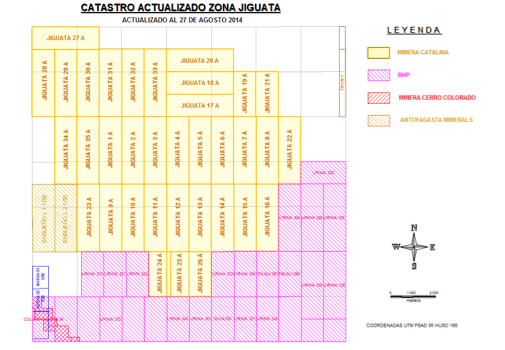
Figure 2: The Jiguata 1A - 35A Concessions.
In August 2013, Catalina registered sixteen new “pedimentos mineros” or exploration concessions (Jiguata 1A-16A) in the Court of Pozo Almonte. These overlaid the original Jiguata 1-16 concessions and have safeguarded Catalina’s mineral rights until at least August 2015. Jiguata 17A -19A were registered during April 2014, replacing the previous Jiguata 17 to 19 concessions.
Following receipt of the results of the geophysical survey at Jiguata, 16 additional pedimentos, Jiguata 20A to 35A, have been added around the original holdings, raising the total ground held to 10,000 hectares and ensuring, where possible, that the areas of main interest are held by Catalina. The concession ownership map (catastro minero) of the Jiguata area shows the layout of the various concessions (Figure 2).
The Jiguata Project
The Jiguata Project was initially targeted as a high-sulphidation epithermal gold project. This was based on its geological setting, the styles of rock alteration observed and the anomalous levels of As, Ba, Bi, Hg, Mo, Pb, Sb and Te in rock chip samples.
Extensive areas show evidence of hydrothermal alteration which, from its form and expression, indicated the presence of an epithermal system with only the highest portions exposed at surface; the main part of the system remaining hidden at depth.
If this interpretation is correct, possible exploration targets would be parallels to buried high sulphidation epithermal deposits, such as Puren, near La Coipa. Although Puren has no surface expression it contains a Measured and Indicated Resource of 1.53 Moz Au equivalent.
High-sulphidation gold projects are genetically related to and often found adjacent to porphyry copper systems. The first indications that a porphyry-style system might also be present at Jiguata came during a brief mapping exercise in 2012 when the presence of high-level porphyry intrusions and porphyry-style veining were noted in outcrop. A subsequent review of previous work by Codelco (the national mining company of Chile) at Jiguata in the mid-1990s provided additional indications of possible porphyry mineralisation. Codelco had drilled two shallow scout RC boreholes and reported several zones of quartz-veinlet stockwork with anomalous Cu-Mo geochemistry.
Analysis of samples from the two holes returned:
- An upper zone of 76 m (52-128 m) with average grades of 251 ppm copper and 107 ppm molybdenum, with maximum values of 5,555 ppm Cu and 250 ppm Mo.
- A lower zone of 163 m (138-301 m) with average grades of 102 ppm copper and 192 ppm molybdenum with maximum values of 1,240 ppm Cu and 933 ppm Mo.
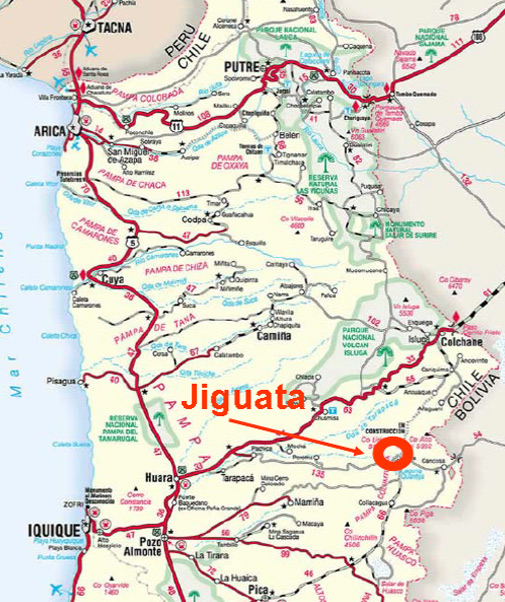
Figure 3: A district-scale satellite image of the Jiguata area.
Neither of these intersections can be classed as economic mineralisation but because the holes ended in ground still hosting anomalous Cu-Mo values it became clear that the development of porphyry-style geology was probably more extensive than first thought and that future exploration should focus on copper porphyry mineralisation in addition to epithermal gold.
It was decided that this could be best achieved by undertaking a broad-scale, reconnaissance geophysical survey using Induced Polarisation (IP) to indicate whether Jiguata is underlain by a porphyry system and to quantify its possible size.
Figure 3 shows:
a) The approximate area of the IP-resistivity survey – the rectangle outlined in green
b) Areas of significant hydrothermal alteration at surface – (the irregular orange outlines)
c) The location of the mineralised porphyry occurrences mapped to date at Jiguata – (the pink/red outlines)
d) Principal district-scale faults (blue) inferred from satellite imagery. These fall into two groups: (a) major, parallel, NW-striking structures and (b) a second set of NE-trending structures. Both sets appear to control the drainage pattern
e) There is a large Miocene age volcanic centre to the NE of Jiguata (the large red triangle) which is separated from Jiguata or controlled by one of the NW-striking faults
The size of the area under investigation is indicated by the scale-bar in the lower left of the image.
Figure 3 also shows that the rocks at Jiguata are affected by hydrothermal alteration (the light-coloured area described in (b) above) and are partly concealed by a widespread younger cover of largely unaltered, darker coloured, sub-horizontally dipping volcanic lava flows. These extend over a large part of the area and occupy the flanks of the major volcanic centre to the NE of Jiguata.
The alteration occurs in an extensive zone (with poorly defined limits), comprises a variety of types and it is exposed locally in valleys in the central and southwestern parts of the project area. The rocks hosting this alteration comprise a sequence of coarse- to fine-grained lithic, crystal-lithic and ash tuffs of inferred dacitic composition, intruded by sill-like and locally more plug-like bodies of feldspar-biotite-hornblende porphyry.
The alteration types are roughly stacked above each other and can be sub-divided into the following assemblages:
- High-level "steam-heated" advanced-argillic alteration (opal-cristobalite-alunite-kaolinite-sulphur)
- Mid-level stratabound chalcedony ± opaline silica horizons (indicative of palaeo-water table levels)
- Deeper, blanket-like, hypogene alunite-bearing (and pyritic) advanced-argillic alteration assemblages partly exposed at the lowest elevations in the base of valleys cutting the area
This zonation indicates that erosion has only reached relatively shallow levels in the hydrothermal system. Argillic alteration of the porphyry bodies indicates some telescoping of the alteration package.
Geological mapping has revealed a broad NW (to WNW) striking structural corridor inferred to strike through the core of the property. This is corroborated by (a) short strike-length mapped fault features, (b) lineaments, (c) brecciation of silicic alteration within the corridor and (d) the preferred NW orientation of several mineralisation features.
The main outcropping porphyry occurrence lies at the intersection of this structural corridor with a valley-forming structure (fault) at almost right angles which strikes to the NE. This intersection correlates with strongly anomalous molybdenum values in rock samples at surface and in the shallow drillholes.
A poorly-defined breccia unit - possibly a diatreme - occurs nearby. The breccia displays advanced-argillic alteration and hosts a range of advanced-argillic and silicic altered clasts, including vein mineralised porphyry. It is developed at the SW margin of the main mineralised porphyry occurrence.
The Recent Geophysical Survey at Jiguata
The geophysical contractor (Argali) completed six lines, one-kilometre apart, varying in length from 7,600 to 9,600 metres for a total of 53.8 line kilometres. Sections of the survey report are reproduced below.
Data from the surveys were presented in a series of sections and plans showing the geophysical response at different elevations and demonstrate the presence of a large chargeability anomaly. The sections also show a variety of other features which vary from section to section, as shown in Figure 7.
Chargeability Anomalies
Strong chargeability anomalies from 20 to 35 mV/V are outlined on all six lines. In several areas, they are fairly close to the surface but in most areas, the anomalies are 100 to 400 m below surface with each line showing relatively-continuous, deep chargeable zones over 5 km wide. The anomalies may be closing to the southwest and northeast on the lines but additional coverage is required to confirm closure. Lines 3000N and 8000N both show strong chargeability anomalies, so the chargeable zone remains open to the SE and NW.
Possible dimensions of the chargeability anomaly (blue outline in Figures 5 and 6) measure approximately 7x7 km. At the relatively shallow depths at 4,100 m elevation, there is close correlation between the porphyry outcrops and the chargeability highs.
Figure 5 shows a plan view of inverted chargeability at a constant elevation of 4,100 m which is approximately from 100 to 400 m deep. The two strongest and shallowest chargeability anomalies occur on Lines 5000N and 6000N and correspond closely with the two known mineralised porphyry exposures. A third strong, shallow anomaly occurs on the southern portion of Line 8000N in an area that has yet to be mapped.
The chargeability plan of the deeper 3,800 m elevation (Figure 6) shows a significant increase in the areal extent of the chargeability anomalies with amplitudes that match the chargeabilities observed over the porphyry mineralisation on the 4,100 m map. Thus, it is possible that porphyry mineralisation is widespread at depth below the post-mineral cover and steam-heated alteration.
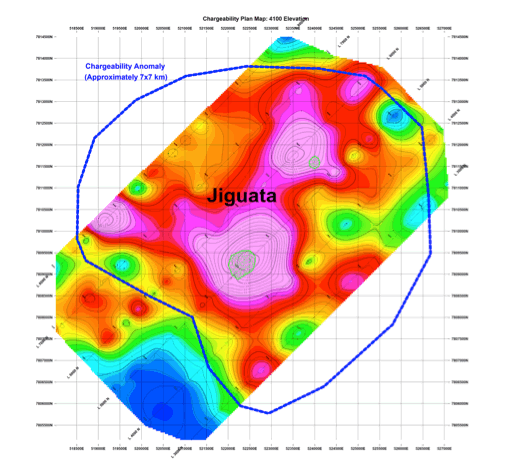
Figure 4: Inverted Chargeability Slice at 4,100 m.
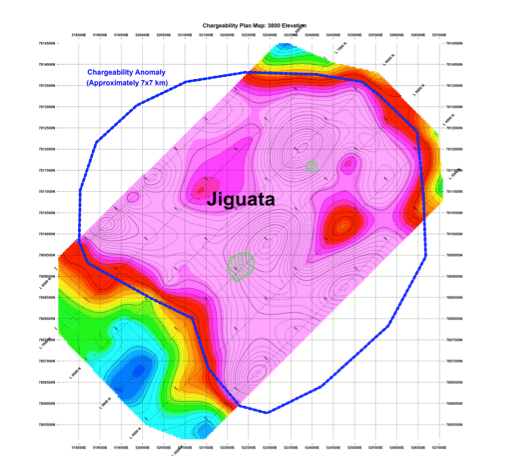
Figure 5: Inverted Chargeability Slice at of 3,800 m elevation.
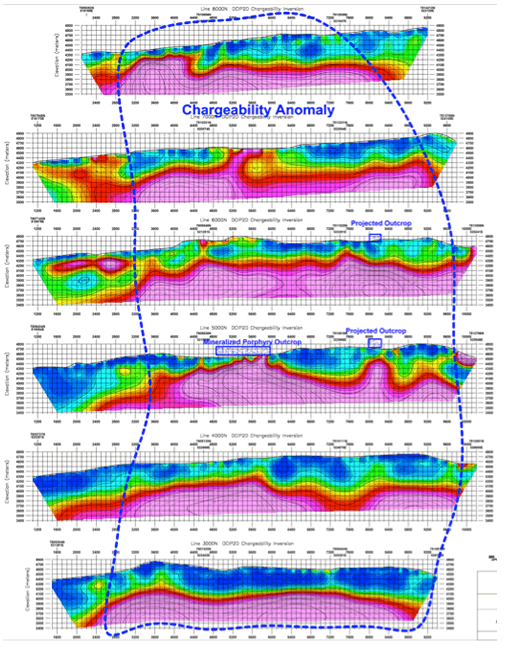
Figure 6: Stacked Inverted Chargeability Sections.
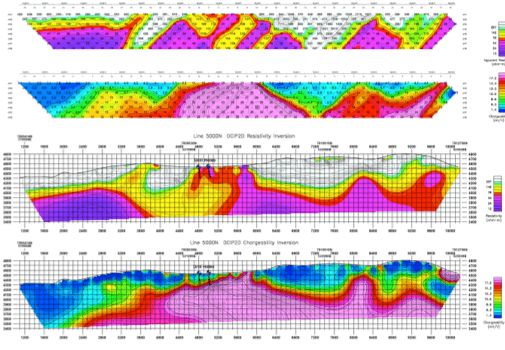
Figure 7: Line 5000N showing pseudosections and inverted resistivity and chargeability sections.
When the inverted chargeability data are viewed in stacked sections (Figure 6), it is apparent that the anomalies extend to at least 800 m - the approximate limit of depth penetration of the survey. Typically, sulphide mineralisation associated with porphyries extends to great depths, so the deeply-rooted anomalies at Jiguata are consistent with expected anomalies over porphyry mineralisation.
Figure 6 also shows several shallower chargeability anomalies with weaker amplitudes and limited depth extension, particularly on the SW portion of lines 5000N, 6000N, and 7000N. These “perched” chargeability zones appear to be caused by a different chargeable source than the stronger, deeper anomalies near the exposed porphyry mineralisation. Epithermal argillic alteration with pyrite could produce such anomalies. Strata-bound epithermal mineralisation or lithologies could also produce such anomalies.
The chargeability and resistivity pseudosection and inverted sections on Line 5000N together with the approximate location of the drill holes are shown in Figure 7. It is apparent that the drill holes are located over the strong chargeability anomaly; however, they do not extend deeply enough to penetrate the strongest part of the anomaly and notably the boreholes only test a small portion of the central anomaly.
Comparison between Jiguata and other porphyry copper deposits in northern Chile
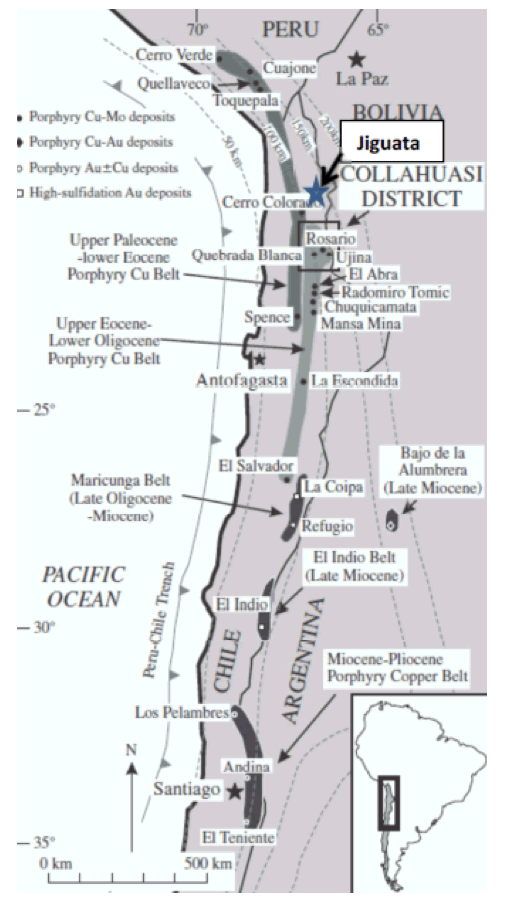
Figure 8: Map showing the Eocene–Oligocene Metallogenic Belt and some of the major mines.
Jiguata lies in prime exploration country at the northern end of the Eocene–Oligocene Metallogenic Belt – one of the most prolific copper mining regions in the world.
The Belt includes over thirty Cu/Mo porphyry deposits containing 220 million tonnes of copper metal and contains world-class mining operations such as Radomiro Tomic, the Collahuasi deposits (Rosario, Copaquire, Quebrada Blanca and Ujina), El Abra, Chuquicamata, Escondida and Zaldivar (Figure 9).
The chargeability and resistivity anomalies at Jiguata are similar to the anomalies observed over the nearby Collahuasi deposits, approximately120-130 km to the south.
The porphyries in the adjacent Collahuasi district all host large chargeability anomalies covering areas from 4 to 6 km in diameter.
The strongest chargeabilities are typically on the outer portion of the anomalous zone and are often considered to be part of the “pyritic halo” that surrounds some types of copper porphyries.
Typically, economic mineralisation is located closer to the centre of the anomalous zone and inside of the strong chargeability associated with the pyritic halo.
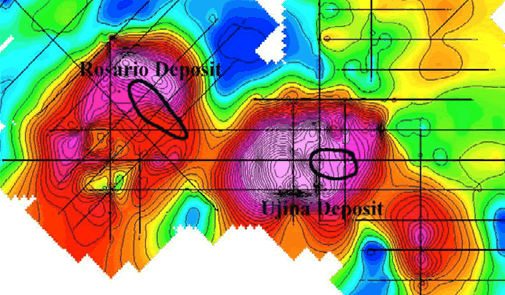
Figure 9: A plan view of the IP data over the Collahuasi-Rosario and Ujina porphyries (From Watts, A., Discovery of the Ujina Cu Deposit, Collahuasi District. Presented at SEG meeting, Oct 2002).
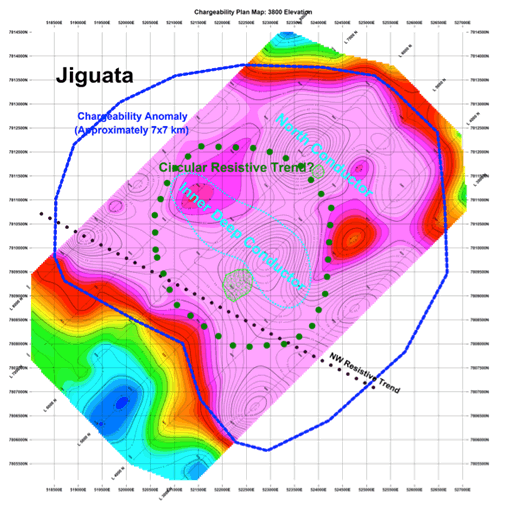
Figure 10: A plan view of the inverted chargeability from Jiguata at 3,800 m elevation.
Not all the chargeability anomalies at Collahuasi carry economic mineralisation. The black outlines in Figure 9 indicate those portions of the chargeability anomalies which carried mineral resources as of 2002. It should be noted that substantial additional mineralisation has since been encountered at depth in both areas, particularly Rosario.
Figures 9 and 10 are shown above at the same scale and indicate that the Jiguata anomaly is somewhat bigger than both the Rosario and the Ujina anomalies but is smaller than the combined anomalies.
The circular resistivity anomaly at Jiguata is approximately the same size as the chargeability anomaly at Ujina. Thus, the dimensions of the Jiguata anomaly are consistent with the dimensions of other porphyry deposits.
Discussion
Strong chargeability anomalies outlined at Jiguata cover a large area measuring at least 6-7 km in diameter. Most of the chargeability anomalies commence at 100 to 300 m depth, typically below a shallow resistive layer that is attributed to post-mineral cover or overprinting by steam-heated epithermal alteration.
The strongest and shallowest chargeability anomalies correlate closely to two outcrops of mineralised porphyry. Chargeability anomalies associated with known or inferred pyrite-bearing epithermal argillic alteration are weak to moderate in comparison to the anomalies near the outcropping porphyry mineralisation.
Consequently, the strong, deep chargeability anomalies at Jiguata may also be associated with porphyry mineralisation.
The two historic drill holes tested one of the porphyry outcrops. These drill holes are located near strong chargeability anomalies; however, these relatively shallow (250m vertical depth) drill holes did not test the main part of the deeper chargeability anomaly, nor did they test the deeper conductive zones. Both holes encountered anomalous gold and copper and high molybdenum values. High molybdenum values are also present at the large porphyries south of Jiguata at Rosario, Ujina, Quebrada Blanca, and Copaquire.
The Jiguata anomalies are considered an excellent target for large-scale Cu-Mo porphyry mineralisation. However, the post-mineral cover and the over-printing epithermal alteration complicate both the chargeability and resistivity anomalies observed. The interpretation of the geophysical anomalies is therefore more complex and ambiguous than in areas hosting only porphyry mineralisation.
The new interpretation of the geology presented above represents a considerable advance in our understanding of the Jiguata area. Furthermore, it greatly expands the geological potential of the Project because, although there is no guarantee at this stage that these newly-identified targets carry economic mineralisation, the focus of Jiguata has shifted from an epithermal gold target to one or more possible copper porphyry targets – an increase of several orders of magnitude.
Follow-up detailed geological mapping and geochemical sampling and additional geophysical surveys are planned. The IP survey has yet to define the full limits of the underlying porphyry intrusives and the purpose of this work will be to define the extent and principal parameters of the potential porphyries before seeking a joint venture partner to assist with the funding of more detailed, later-stage exploration.
The geophysical survey has demonstrated clearly that IP reflects the underlying geology and additional surveys, on more closely-spaced lines, will be valuable in defining targets for future scout drilling.